3.2 The cellular Basis of life
As mentioned above there is a cellular basis to all life. All living things are either single cells themselves or multicellular (made up of cells).
Why are cells the fundamental unit of life?
All the processes that characterise life – metabolism, homeostasis, growth and reproduction – can only exist within the confines of cells. So, defining a perimeter and confining all processes within it is a fundamental aspect of the organisation that allows life to exist. We call this aspect of organisation compartmentalisation. All cells define their perimeter by a cell membrane. Without an intact cell membrane, the cell has no organisation and cannot live. Many cells, including our own, exhibit further internal compartmentalisation and confine specialised processes and functions to areas defined by internal membranes.
Things common to all cells (suggesting a common ancestry)
Although all life is either cells or made up of cells, the cells themselves show incredible diversity in size, shape and function. However, before focusing on the differences between cells it is worth thinking about what they have in common. There are four components that all cells share:
- All cells have a plasma membrane. This is an outer covering that separates the cell’s interior from its surrounding environment. The membrane is primarily composed of phospholipids. It not only defines the perimeter of the cell and contains the cell’s components, it also provides a way for the cell to interact with its environment in a controlled manner. The plasma membrane allows the selective passage of molecules into or out of the cell.
- All cells have a cytoplasm. The cytoplasm is a gel-like fluid inside the cell (comprised of water, salts and typically a surprisingly high concentration of macromolecules) that provides a medium for chemical reactions. Diagrams of cells often give the wrong impression of cytoplasm as a simple bag of water. In reality, the cytoplasm is crowded with macromolecules. The cytoplasm of the bacteria Escherichia coli contains 300–400 mg/ml of macromolecules (see Figure 3.7). This makes the cytoplasm viscous and affects the properties of all of the components in a cell. For instance, the high concentration of macromolecules reduces the volume of water available for other molecules, effectively increasing their concentration.
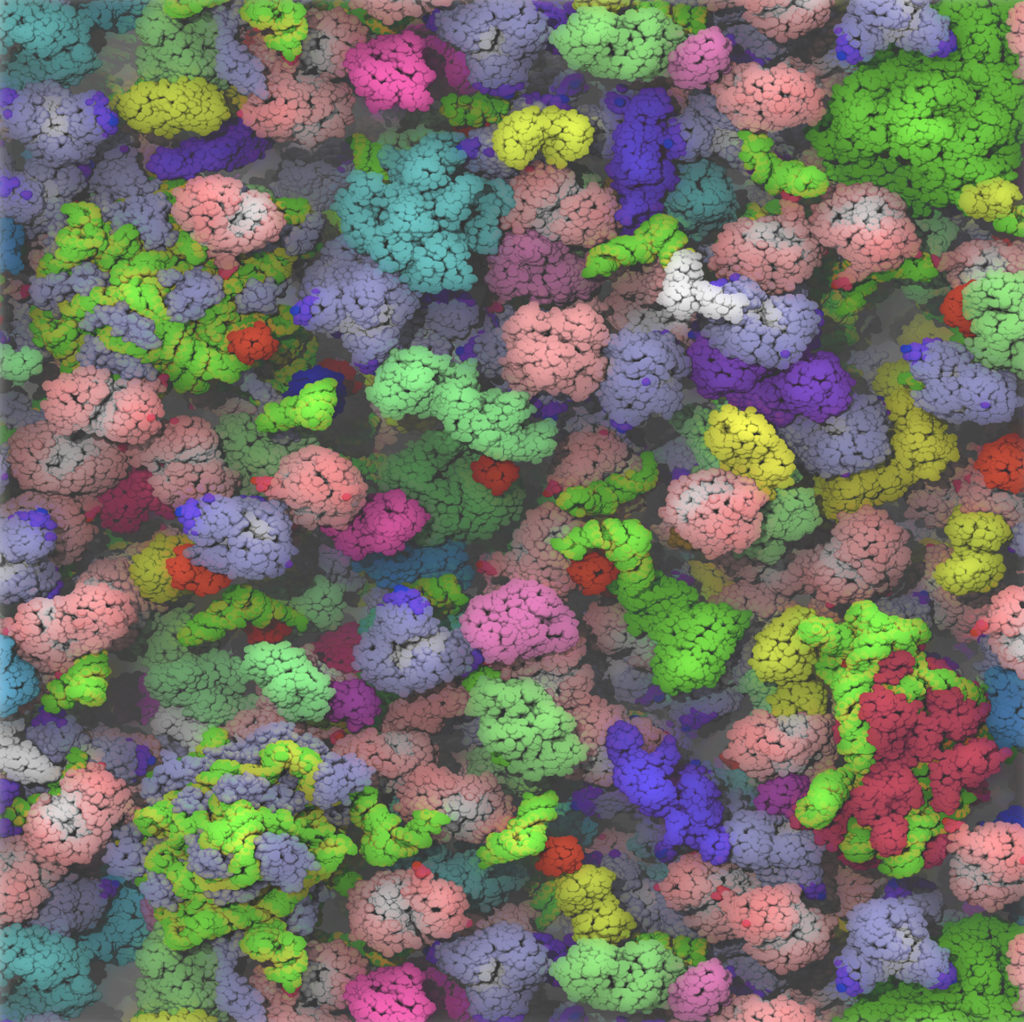
- All cells use DNA as their genetic material. In fact, all cells store their hereditary information in the same form of double-stranded DNA using the same four types of nucleotide monomers (adenine, thymine, cytosine and guanine or A, T, C and G). All cells use the same mechanism to replicate their DNA, when they grow and reproduce, of semi-conservative replication where each strand acts as a template for the new strand. All cells transcribe portions of their DNA into RNA, which acts as an intermediary species for the production of proteins (some RNA is functional in its own right; e.g. ribosomal RNA). And all cells use the same four types of nucleotide monomers (adenine, uracil, cytosine and guanine) to make the RNA polymer. Furthermore, the genetic code (the set of rules defining how the four-letter code of DNA is translated into the 20-letter code of amino acids making up proteins) is nearly universal with only a few exceptions reported (see Chapter 4).
- All cells contain ribosomes, which are the sites of protein synthesis. Often the ribosome is described as an organelle, but it is not enclosed by a membrane and better thought of as a macromolecular machine. Ribosomes are composed of RNA (approximately 60%) and protein (approximately 40%). While ribosomes can differ between species, the core of the ribosome and how it functions is the same in all living systems.
These shared characteristics of all cells, particularly the use of the same genetic material, a near universal genetic code and the ubiquitous use of ribosomes for protein synthesis, point to all life on Earth sharing a common ancestor. This last universal common ancestor (LUCA) of all cells is thought to have lived approximately 4 billion years ago.
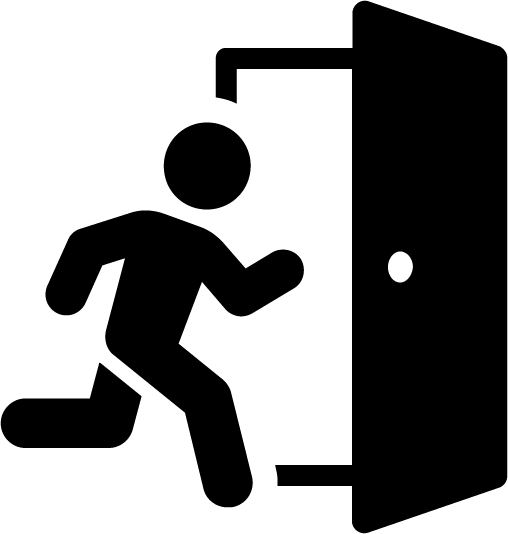
The evolutionary relationship between organisms is known as phylogeny. Since all life uses the same hereditary DNA molecule and common processes (e.g. protein synthesis), it is possible to use differences in the DNA sequences of common genes to establish how closely related species are to one another. Since cells all use ribosomes, and all for the same purpose of protein synthesis, the structure of ribosomes changes very slowly over evolutionary time. This makes them a useful way to measure evolutionary relationships over long periods. Figure 3.8 shows a phylogenetic model (a phylogenetic tree) based on comparing the sequences of ribosomal RNA (rRNA) from many species. This tree places all life in three distinct domains – bacteria, archaea and eukarya – with our last universal common ancestor at the bottom. The lineage of eukaryotes is more complicated as they are almost certainly the result of two or more cells merging in a process called endosymbiosis (see Chapter 4).
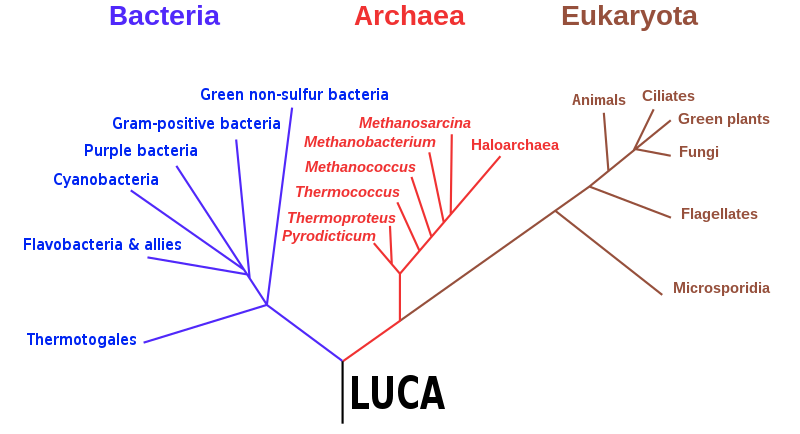
Despite this lineage as describe, cells are classified into two broad groups: prokaryotes and eukaryotes. Bacteria and archaea are all prokaryotes. Typically, prokaryotes are all unicellular organisms (though some species are capable of forming colonies). Eukaryote cells are in the domain eukarya. Eukaryotic cells are much larger than prokaryotic cells (typically 10 to 100 times bigger) and can be either unicellular or multicellular.
However, the major difference between eukaryotes and prokaryotes is that eukaryotic cells have internal compartmentalisation and prokaryotes do not. The internal compartmentalisation of eukaryotes consists of internal membrane structures that enclose particular cell constituents and processes. These structures are known as organelles.
The most obvious difference in this respect is the presence of a nucleus in eukaryotes and the absence of one in prokaryotes. The nucleus is a membrane-bound organelle that functions as the site of DNA storage of DNA. Prokaryote literally means ‘before the nucleus’ (pro means before and karyon means nut or kernel).
Eukaryotic cells have several other membrane-bound organelles located within their cytoplasm that are not found in prokaryotic cells. The major ones are the mitochondria (an important site for energy production); rough and smooth endoplasmic reticulum (an interconnected network of membrane-enclosed tubules that transport synthesised proteins); Golgi complex (for protein secretion); and in the case of plant cells, chloroplasts (which conduct photosynthesis).
Compartmentalisation in eukaryotes has some functional implications. Due to compartmentalisation, cell division in eukaryotes is a much more complicated and regulated process (mitosis) while prokaryotes can divide by simple binary fission. The presence of a nucleus in eukaryotes means that transcription (which occurs in the nucleus) is decoupled from translation (which occurs in the cytoplasm). In prokaryotes these two processes occur simultaneously.
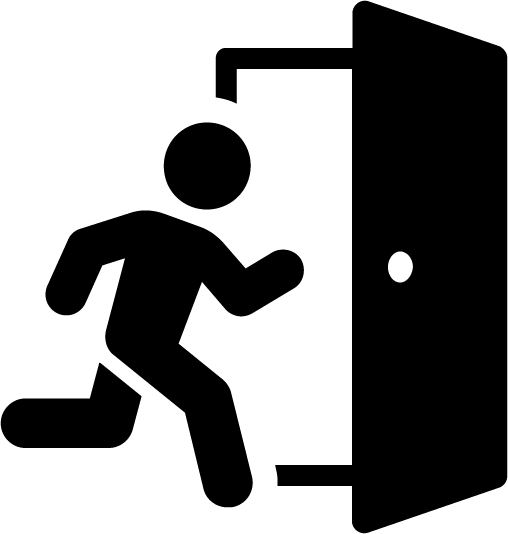
Discussion questions
- What happens to an organism that reaches a state of thermodynamic equilibrium?
- Many chemical reactions in the cell are energetically unfavourable, such as synthesising nucleic acid polymers from nucleotide monomers. How do unfavourable chemical reactions occur in the cell?
- Exobiologists are researchers who seek life outside the Earth. Therefore, they need a definition of life that determines what they will look for. In 1994, NASA scientists involved in their exobiology program proposed the following definition: ‘Life is a self-sustaining chemical system capable of Darwinian evolution’.
- This appears to be a simple definition. Which of the characteristics common to all life does it support?
- Can you think of any entity generally agreed upon to be living that is excluded by this definition?
- Do you think that NASA’s definition is practical for exobiologists seeking life outside the Earth? In 2013, Azua-Bustos and Vega argued that regardless of the types of life on Earth or that might be found elsewhere in the universe, all life should share the attribute of decreasing internal entropy at the expense of free energy obtained from their surroundings. How could this be practically measured?
- A human baby acquires, on average, approximately 70 random mutations to its genomic DNA compared to its parents. Why do the vast majority of these mutations have no phenotypic effect?
- Homeostatic mechanisms are controlled by negative feedback loops. What are positive feedback loops? Can you think of one example of a positive feedback loop that would be deleterious to an organism and one that might be beneficial to an organism?
- Explain why the following statement is incorrect.
‘As animals evolve, they adapt to their environment. If they encounter a problem such as increased toxic chemicals in their environment, they evolve new mechanisms to mediate the toxicity by changing their DNA. Changing their DNA ensures that their offspring will be resistant too.’