2.4 Advice for studying biology at the molecular level
Yes, biochemistry and molecular biology at times can be challenging, but it is not all bad news! There are a few things to note and a few approaches you can follow that should make things easier than they initially appear or pay dividends in the long run.
All extant life is related. This is a very good thing!
All extant life (living organisms which exist now on Earth) has a common ancestor. The evidence for this is presented in Chapter 3. This means that even with all of the amazing diversity of life around us, because all living things are related, they work in fundamentally the same way at the molecular level. For example, all life uses DNA to store genetic information and codes it essentially in the same way, and all life uses the same or similar basic molecular building blocks and organises them in the same way. This means that once you understand a few basic principles, you will be able to apply this knowledge to all living things. Imagine how difficult it would be if this were not the case, and we had to learn everything from scratch when we moved on to a new organism!
Finding the patterns
Biology (and in fact all science) is concerned with discovering relationships between variables. That is, it is about discovering trends and patterns. The most useful discoveries in biology are those that are explanations for general phenomena. Similarly, when you are learning a discipline, it is most useful to learn the principles that have the broadest applicability.
When you first learn biology at the molecular level it might seem that there is a bewildering amount of information to learn. There is a lot! But you will also realise that many processes work in a similar way or even use the same or very similar components.
In his essay ‘Evolution and Tinkering’, published in Science in 1977, the French biologist and Nobel laureate, François Jacob described evolution as a ‘dogged tinkerer’. What he meant by this is that evolution should not be compared to engineering, where a new function is designed and created from scratch. Rather, the process is similar, in Jacob’s words, to a ‘tinkerer [who] picks up an object which happens to be in his stock and gives it an unexpected function. Out of an old car wheel, he will make a fan; from a broken table, a parasol.’
We often mention adaption when discussing evolution. This is where changes to a population or species occur due to selective pressure of the environment (see Growth, reproduction and evolution in Chapter 3). However, there is another important process called exaptation. This is where a trait can shift in function during evolution. That is, like how the tinkerer works, the trait is given an unexpected new function.
This might be difficult to understand at this stage but will become clearer after you read chapters 3 and 4. At the moment just understand that because evolution will reuse and repurpose biological components for new functions, you will see that similar mechanisms occur in different cellular processes. The same molecule or mechanism will be seen in different pathways, and different complex signalling or biosynthetic pathways will contain the same modular components.
For example, the molecule adenosine is a component of DNA, RNA, adenosine triphosphate (ATP), coenzyme A, nicotinamide adenine dinucleotide (NAD), flavin adenine dinucleotide (FAD) and cyclic adenosine monophosphate (cAMP). These participate in many different cellular processes but once you first learn about adenosine you will much more easily see how it fits into all these different places.
Another example is G-protein coupled receptors, a large superfamily of cell surface receptors that have incredibly diverse functions (photoreception, taste, pain and smell; they also mediate cell recognition, metabolism and growth) despite having a very similar structure and common mechanism of action. All G-protein coupled receptors share two properties – they have seven transmembrane domains, and they interact with specialised proteins (called G proteins) to influence intracellular pathways after binding extracellular signals (see Figure 2.4).
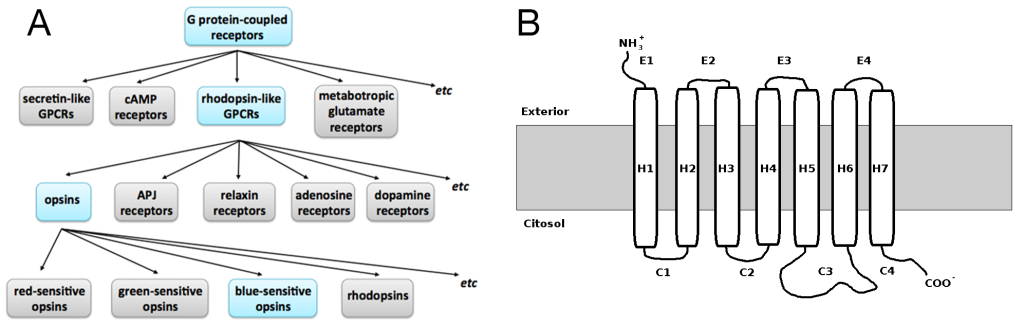
Figure 2.4 B: Despite the diversity of functions this entire family of proteins share a common canonical structure containing seven transmembrane domains and a common mode of action elicited through a coupled g-protein. Image: ‘GPCR’ by Retama from Wikimedia Commons used under CC BY-SA 3.0.
Getting to grips with the language
Part of the challenge you will face initially with biochemistry is learning new terms. The American author and educator Neil Postman said[1]:
Biology is not plants and animals. It is language about plants and animals …
This quote highlights the importance of language when learning about biology. It is not just about learning new words but also how to use words you already know in a biological or scientific context. Without understanding language and naming conventions to a level where you use them in your speech and writing, your learning will be limited. This is not trivial – in science classes the number of new terms can be comparable to foreign language classes!
In addition, there are two ways in which language is confusing in biochemistry:
- Words are often borrowed from everyday English and repurposed. This can make it difficult as you will need to re-learn a word you know but in its scientific context.
For example, when we speak about tissues in everyday language, the brand Kleenex may come to mind. However, in cell biology, tissues refer to a level of organisation in multicellular organisms consisting of a group of either functionally or structurally similar cells plus their surrounding intercellular material.
Similarly, words like transcription or translation have a meaning in everyday writtend and spoken language. However, in molecular biology they specifically refer to the transfer of genetic information in living organisms.
With this kind of terminology, the key is to ensure that you understand the processes they are associated with. Understanding them in context is the key to not confusing their counterpart meaning in everyday English.
- Many words are long and complex and have origins from other languages, principally Greek and Latin. These, just because of their unfamiliarity and length, will be difficult to read and intimidating to say aloud.
Becoming fluent in the language of biology
When learning anything complex it is a good idea to break it down into smaller parts to make it more accessible.
Scientific words often have three parts – a prefix, a root and a suffix, as shown in the following table.
Prefix | Root | Suffix |
Appears at the beginning of a word. Many are used frequently, so you will eventually learn their meaning. | The root of a word often has a standalone meaning. These often have a Greek or Latin origin. | Appears at the end of a word to provide additional meaning. |
hypo – below
hyper – above cyclo – ring poly – many endo – within exo – outside of |
iso – equal
allo – other chloro – green cyte – cell |
-phillic – love, affection
-phobic – hate, fear -lysis – breakdown -some – body -oma – abnormal, cancer |
You will be surprised how often the same prefix, root or suffix will appear in different words. Once you learn a few, things will start to get easier. For example, the Greek word therm means ‘heat’. You will find this word in a large family of scientific terms, including exothermic, thermogenesis, thermoregulation, isothermal, ectotherm, thermodynamics, hypothermia and many others.
Knowing the word origin (etymology) of a term can also help to identify its likely meaning and make it easier to understand in biochemistry.
For example, the word eukaryote is a noun describing a cell containing a nucleus. It is derived from the prefix eu– (from Greek, meaning good, well or true), the root kary (from the Greek word karyon, which means kernel or nut but now indicates the cell nucleus) and the suffix –ote (a singular form of the Greek-derived word osis, which means process or state).
Resources
There are many useful websites that can help you with understanding the etymology of words.
- Online Etymology Dictionary
- The Free Dictionary also contains a thesaurus, medical dictionary and many other features. You can listen to a pronunciation of almost all words in the dictionary.
When learning new words, writing them in a sentence of your own construction can help integrate them into your vocabulary faster. Also say them aloud. Try not to be intimidated by their length – most of the time they will be phonetic (sound the way they are spelled). So just break them down into their component parts and at first say them slowly until you get used to pronouncing them! When you can confidently say them in your head, reading will be much easier, and your learning will rapidly accelerate.
Learning Should be Active
Despite the importance of concepts (see below) some things just need to be committed to memory. However, memorisation is far more effective if it is done by active learning rather than passive. If you simply read text or watch lectures the information will simply wash over you. However, if you write things down (preferably by hand), create diagrams and flowcharts and constantly check your knowledge by answering questions you will be far more likely to successfully commit important information to memory. Give yourself plenty of time to study so you can leave enough time between knowledge checks, so you know you are not just testing your short-term memory.
Concepts rule!
The reason for learning anything in the first place is to be able to apply that knowledge to novel situations. This means that it is always much more important to learn concepts rather than isolated facts. Biochemistry and molecular biology as disciplines are no different. This does not mean that facts are unimportant! It is just that understanding ideas and concepts will help you learn facts faster. Learn to understand rather than just simply memorise.
An excellent example of the importance of concepts versus facts is the development of the periodic table of the elements. The Russian chemist Dmitri Mendeleev set out to order the known chemical elements in the 1860s (at the time only 63 elements were known). He noticed that there was a correlation between atomic weight and the chemical properties of elements. This led to ordering elements by increasing atomic weight but also into horizontal rows called periods, where each period number indicates the number of electron orbitals for the elements in that row. As elements were discovered they were added logically to the gaps in the table.
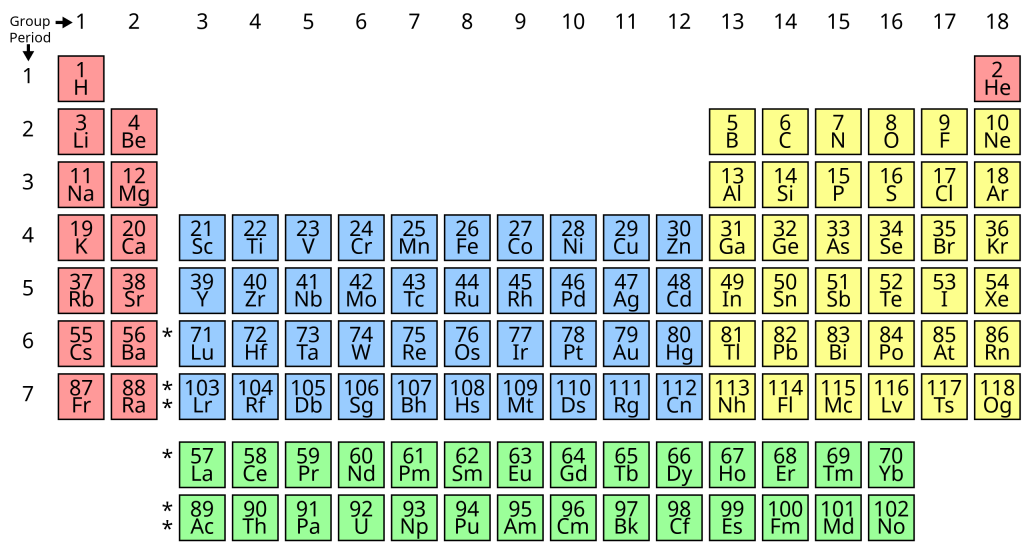
For students of chemistry (and biochemistry!) where knowledge of the elements is important, it is far better to understand the main concepts underlying the periodic table than to memorise the chemical properties of individual elements.
Conceptual learning is important but there is a hierarchy of concepts too. Some are more important than others in the sense that by learning them they will allow you to connect many different concepts. We call these threshold concepts, and mastering them is the purpose of this e-book.
Mastering the threshold concepts
A threshold concept refers to one of the core concepts in a subject whose understanding is key to transforming the way you understand the subject as a whole. To understand what we mean by a threshold concept, consider this example from the unrelated discipline of history.
When you begin learning history you may focus on names and dates and accept an initial historical account you read to be accurate. However, as you study in more depth, you begin to understand that historical accounts can be biased, and there can in fact be different competing historical accounts. Often there is no clear definition of why something happened or even a definitive account of what actually happened! The threshold concept in this case is that there is an interpretive aspect to history. This is transformative as once you understand it, you will never read history in the same way again.
So, threshold concepts are transformative (create a significant shift in your understanding), probably irreversible (once learnt are difficult to unlearn), integrative (allow you to make links between different topics) and potentially troublesome (difficult to initially grasp and often counterintuitive).
The focus of this resource is to help you understand the key threshold concepts in biochemistry and molecular biology. These have been identified by research[2] funded by the American National Science Foundation. Key threshold concepts critical for biochemistry were identified in five major areas:
- The central importance of the theory of evolution to all biological sciences
- Matter and energy transformation
- Homeostasis, control and regulation
- Biological information
- Macromolecular structure and function